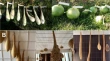
Overview
Call for Papers: Food, Metabolism, and Nutrition in Kidney Disease: Implications and Perspectives
Nutrire is an international journal that brings together research in Nutrition and Food Science.
- Fully indexed in Scopus with all articles published since 2016 included in the database
- No article processing charge for authors, unless opting for open access
- The official journal of the Brazilian Society for Food and Nutrition
- Nutrire is an online-only journal and its print ISSN (1519-8928) is ceased
- Editor-in-Chief
-
- Marcos Minicucci,
- Olga Amancio
- Submission to first decision (median)
- 4 days
- Downloads
- 144,962 (2023)
Societies and partnerships
Latest articles
Journal updates
-
[Call for Papers] Food, Metabolism, and Nutrition in Kidney Disease: Implications and Perspectives
Check Aims and Scope, focus areas, deadline and more.
Journal information
- Electronic ISSN
- 2316-7874
- Abstracted and indexed in
-
- Baidu
- CAB Abstracts
- CLOCKSS
- CNKI
- CNPIEC
- Chemical Abstracts Service (CAS)
- Chinese Academy of Sciences (CAS) - GoOA
- Dimensions
- EBSCO
- EMBASE
- Gale
- Google Scholar
- IFIS Publishing
- Naver
- OCLC WorldCat Discovery Service
- Portico
- ProQuest
- SCImago
- SCOPUS
- Semantic Scholar
- TD Net Discovery Service
- Wanfang
- Copyright information